Raymarine SeaTalk NG
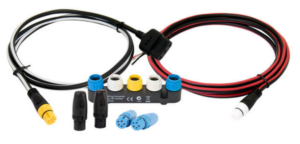
Fig.: SeaTalk NG connection technology
With SeaTalk NG there is a more powerful successor to SeaTalk, which is based on the CAN bus and has limited compatibility with NMEA2000. With the fairly clear and color-coded connection technology of SeaTalk NG, Raymarine has managed to provide backwards compatibility with SeaTalk, which also supports older devices. The disadvantage, however, is the high price for the proprietary connection technology.… Continue reading
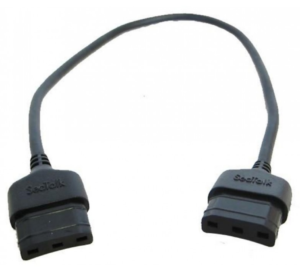
Fig.: SeaTalk connection technology
SeaTalk is a Raymarine proprietary serial network protocol developed in the 1980's and used for the transmission of navigation data and other information on boats. The data is transmitted as compact binary data. SeaTalk uses a single wire connection as the data line for serial data transmission, meaning they are both sent and received bit by bit over a single wire.… Continue reading
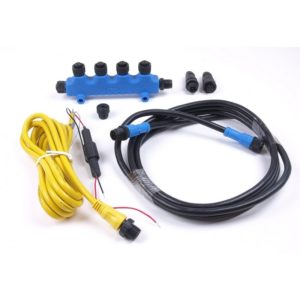
Fig.: NMEA2000 connection technology (Busse yacht shop)
NMEA2000 (National Marine Electronics Association 2000) is a bus system for communication in boats. It was designed to provide a uniform interface for transferring data from electronic devices on boats.
operating principle
NMEA2000 is a bus system designed to transmit data between electronic devices on boats.… Continue reading
First of all, a few important notes that you should definitely pay attention to.
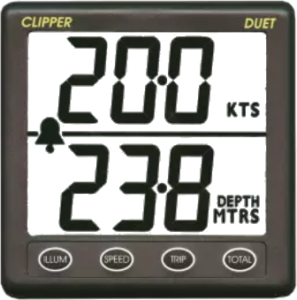
Fig.: NASA/Clipper Duet Sonar/Log
This article is about how to convert a NASA/Clipper Duet echo sounder/log so that the device outputs NMEA2000 data. This document is based on the DIY project by Sönke and was supplemented with some additional information. The NASA/Clipper Duet echo sounder/log is actually a device without a bus connection and works independently with two separate sensors.… Continue reading
First of all, a few important notes that you should definitely pay attention to.
Raspberry Pi
The main hardware base for AVnav and OpenPlotter is the Raspberry Pi. It is a small computer in credit card format. It is available in several variants, although we are only concentrating on the Raspberry Pi 4B here. Of the older models, only the Raspberry Pi 3B is still interesting.… Continue reading
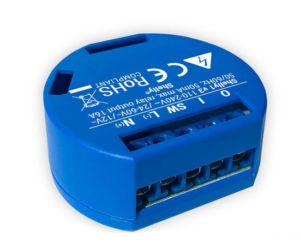
Fig. Shelly 1
Anyone who anchors is obliged to set an anchor light so that you can be recognized on the open water surface in the dark. Typically, the light is switched on manually at dusk and off again at sunrise. There is no problem switching it on. But often you forget to switch it off and the anchor light is then permanently on and consumes electricity unnecessarily.… Continue reading
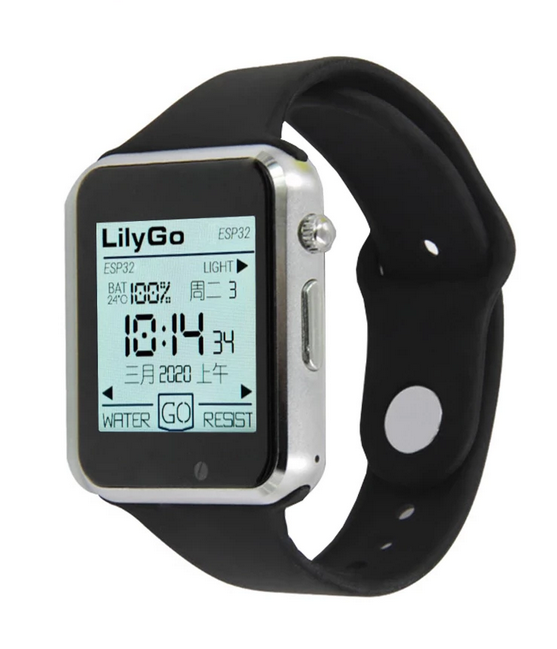
Fig. Lilygo T-Watch 2020 (Lilygo)
Jan Dytrych has started a software project to be able to display data from SignalK and receive alarms with a smart watch. Not just any common clock under Android is used as a smart watch. He uses the smart watch Liligo T Watch 2020. The special feature of this clock is that it has an ESP32 installed as a processor and can be controlled via the Arduino IDE or… Continue reading
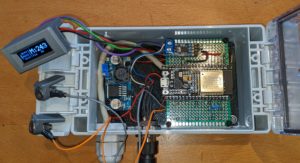
why
I've always found the idea of not only showing the course on my plotter when the boat is moving, but also when it's bobbing along quietly, I've always found it exciting.
After searching the I-Net for different manufacturers, it quickly became clear that I might not need an e-compass on board at current prices.… Continue reading